|
Fungal Diversity and Bioremediation
Dr. S. B. Sullia
Department of Microbiology & Biotechnology
Bangalore University,
Bangalore-560056
The abundant variation in the types of all living organisms taken together in any geophysical area is called biodiversity. With reference to large organisms (plant, animals etc.) the distinctive morphological and anatomical features visible to the naked eye enable us to identify the different genera and species and. therefore, we can easily assess the extent of their diversity. However, most fungi are not visible to the naked eye and they need to be studied under the microscope. The culturable fungi can be identified provided they sporulate but the biotrophs which are not amenable to culturing in growth media go unnoticed. It is, therefore, imperative that there is a huge gap in our knowledge of the diversity of all microbes including fungi. Fungi are better known than bacteria , viruses and other smaller forms of life such as viroids. There are about 72,000 named species and new species are being added at the rate of about 1500 each year. The total number of fungal species both known and unknown is estimated at 250,000 (Hawksworth & Kirsop ,1988; Heywood, 1995). The 72,000 known species exist in different culture collection centres in the form of several subspecies, pathovars and strains, the total cultures going up to 170,000 (Bennett & Faison, 1997). However, several described strains are not deposited in culture collection centres and are not accessible.
Functional Diversity
While dealing with microorganisms it is appropriate to give equal emphasis to structural (morphological) and functional diversity. Based on their activities, the fungi can be grouped as cellulase producers, lignin degraders, nitrate reducers, pectin degraders, P-solubilizers, iron chelaters, Penicillin producers &c. The common traits between a set of fungi implies the presence of certain common gene clusters which have value in a particular ecological niche (Watts et. al., 1999).
Habitat Diversity
The mycoflora of unique ecological niches have some common features and it will be very rewarding to explore fungal diversity in habitats such as thermophilic environments, e.g., hot springs, thermal vents, sun-heated soils, compost pits, self-heated coal refuse piles, steam line discharge sites, etc. The thermophilic fungi and bacteria grow at temperatures between 40-60 C are the sources of thermostable enzymes. Other ecological niches to be explored are western ghats, the Himalayan ranges, marine ecosystems, mangroves, coral reefs, sand dunes, industrial effluent contaminated soils, ant hills, refineries, activated sludge, insects and several other natural sources. Endophytes of plants and animals are very meagerly understood.
Bioprospecting
The process of looking for new forms of life and/or their products is called Bioprospecting. The techniques of fungal exploration have remained by and large conventional. The methods have mainly included the use of different recipes for culture media along with techniques such as dilution plating, soil plating, leaf shred inoculation &c. Direct incubation and baiting techniques have also been in use. The emphasis is now being laid on enrichment techniques wherein the medium used mimics the natural habitat in nutritional status. One can isolate nitrate reducers using a medium enriched with nitrate and minimal carbon source. Reduction of carbon source is also a means of keeping the contaminants away. Addition of antibiotics in enrichment cultures is another way of keeping away the weedy fungi and bacteria. Enrichment cultures have often been used to isolate fungi capable of degrading certain specific xenobiotics. The xenobiotic in question may be used as the sole carbon source in the medium so that only the fungi with degradative capacities will grow. In liquid culture, competition for the particular substrate will lead to the enrichment of the microbial strain that is able to grow faster than others. One flaw in the enrichment technique is that it leads often to growth of bacteria even when fungi with good potential are present. It is because of the capacity of bacteria to grow faster than fungi. Besides, some fungi may not utilize the degraded product as the sole source of carbon or as a supplementary source of carbon, as in the case of the white rot fungi (Jefries et al., 1981). It may be therefore necessary to use bacteriostatic compounds and supplementary carbon sources to isolate xenobiotic degrading fungi from a natural source.
When it is not possible to grow a fungus by any means, isolation of the gene pool from the environment may be very rewarding. Environmental DNA is now being harnessed for commercial production of certain substances without the need to grow the actual microorganism. The gene of interest separated from environmental DNA can be tagged onto a vector and cloned into a suitable expression host to yield the product. More work has been done this area with bacteria than fungi.
Bioprospecting is important because fungi and other microorganisms are the largest future source for various products and processes. They are of critical importance to the sustainability of life in nature because they are involved in the recycling of elements and in producing and consuming gases such as oxygen and carbon dioxide so important in maintaining our climate. Fungi have been employed for the industrial production of compounds of intrinsic value such as antibiotics, enzymes, hormones, immunosuppressants, food additives and colorants. They have also been employed as biopesticides and biofertilizers. They have been the sources of microbial protein and oil and animal feed. Edible fungi have been known as long as human civilization. The exploration of the extent of fungal diversity on planet earth is indeed a stupendous, but surely a very rewarding task.
Bioremediation
Bioremediation is a pollution control technology that uses biological systems to catalize the degradation or transformation of various toxic chemicals to less harmful forms. The general approaches to bioremediation are to enhance natural biodegradation by native organisms (intrinsic bioremediation), to carry out environmental modification by applying nutrients or aeration (biostimulation) or through addition of microorganisms (bioaugmentation). Unlike conventional technologies, bioremediation can be carried out on-site. Bioremediation is limited in the number of toxic materials it can handle (Hart, 1996), but where applicable, it is cost-effective (Atlas & Unterman, 19977).
Biodegradation, mineralization, bioremediation, biodeterioration, biotransformation, bioaccumulation and biosorption are some terms with minor differences but often overlappingly used. Biodegradation is the general term used for all biologically mediated break down of chemical compounds and complete biodegradation leads to mineralization. Biotransformation is a step in the biochemical pathway that leads to the conversion of a molecule (precursor) into a product. A series of such steps are required for a biochemical pathway. In environmental terms, it is importance whether the product is less harmful or not (Bennett & Faison, 1997). Biodeterioration refers usually to the breakdown of economically useful compounds but often the term has been used to refer to the degradation of normally resistant substances such as metals, plastics, drugs, cosmetics, painting, sculpture, wood products and equipment (Rose, 1981). Bioremediation refers to the use of biological systems to degrade toxic compounds in the environment. Bioaccumulation or biosorption is the accumulation of the toxic compounds inside the cell without any degradation of the toxic molecule. This method can be effective in aquatic environments where the organisms can be removed after being loaded with the toxic substance.
The fungi are unique among microorganisms in that they secrete a variety of extracellular enzymes. The decomposition of lignocellulose is rated as the most important degradative event in the carbon cycle of earth (Bennett & Faison, 1997). Enormous literature exists on the role of fungi in the carbon and nitrogen cycles of nature (Frankland et al., 1982; Cooke & Rayner, 1984; Carroll & wicklow, 1992). The role of fungi in the degradation of complex carbon compounds such as starch, cellulose, pectin, lignin, lignocellulose, inulin, xylan, araban etc., is well known. Trichoderma reesei is known to possess the complete set of enzymes required to breakdown cellulose to glucose. Degradation of lignocellulose is the characteristic of several basidiomycetous fungi.
Fungi in bioremediation
Fungi are good in the accumulation of heavy metals such as cadmium, copper, mercury, lead and zinc. Systems using Rhizopus arrhizus have been developed for treating uranium and thorium (Teen-Seers et al., 1984).
The ability of fungi to transform a wide variety of hazardous chemicals has aroused interest in using them in bioremediation (Alexander, 1994). The white rot fungi are unique among eukaryotes for having evolved nonspecific methods for the degradation of lignin; curiously they do not use lignin as a carbon source for their growth (Kirk et al., 1976). Lignin degradation is, therefore, essentially a secondary metabolic process, not required for the main growth process. Lamar et al. (1993) compared the abilities of three lignin-degrading fungi, Phanerochaete chrysosporium, P. sordida and Tramates hirsuta to degrade PCP (Pentachlorophenyl) and creosote in soil. Inoculation of soil with 10% (wt/wt) Phanerochaete sordida resulted in the greatest decrease of PCP and creosote. P. sordida was also most useful in the degradation of PAHs (Polycyclic aromatic hydrocarbons) from soil. Davis et al., (1993) showed that P. sordida was capable of degrading efficiently the three ring PAHs, but less efficiently the four-ring PAHs.
Phanerochaete chrysosporium has been shown to degrade a number of toxic xenobiotics such as aromatic hydrocarbons ( Benzo alpha pyrene, Phenanthrene, Pyrene) chlorinated organics (Alkyl halide insecticides, Chloroanilines, DDT, Pentachlorophenols, Trichlorophenol, Polychlorinated biphenyls, Trichlorophenoxyacetic acid), nitrogen aromatics ( 2,4-Dinitrotoluene, 2,4,6-Trinitrotoluene-TNT) and several miscellaneous compounds such as sulfonated azodyes. Several enzymes that are released such as laccases, polyphenol oxidases, lignin peroxidases etc. play a role in the degradative process. In addition, a variety of intracellular enzymes such as reductases, methyl transferases and cytochrome oxygenases are known to play a role in xenobiotic degradation (Barr & Aust, 1994).
Phanerochaete chrysosporium has been shown to effect the bioleaching of organic dyes ( Nigam et al. ,1995). Pauli ollikka et al. (1993) have also shown the decolorization of azo-triphenyl methane dyes by lignin peroxidase produced by P. chrysosporium. Sami and Radhaune (1995) have demonstrated the role of lignin peroxidase and manganese peroxidase from P. chrysosporium in the decolorization of olive mill wastewater. The work carried out in our laboratory ( Asoka, Manjunath & Sullia, 2000; Asoka, Geetha & Sullia,2002 ) has shown that Phanerochaete chrysosporium and microbial consortia were effective in color removal from textile dye effluents The fungus caused 80% decolorization in broth containing 2.5% of effluent. There was reduction in BOD and COD values. A local isolate of Fusarium sp., caused various degrees of decolorization ranging from 35 to 85 %.
Among the fungal systems, Phanerochaete chrysosporium is emerging as the model system for bioremediation. The basidiomycetous fungus Pleurotus ostreatus has been shown to produce an extracellular hydrogen peroxide dependent lignolytic enzyme that removes the color due to remozol brilliant blue. Oxidative enzymes play a very major role in biodegradation. Other fungi that can be used in bioremediation are obviously the members of Zygomycetes e.g., the mucoraceous fungi and the arbuscular mycorrhizal fungi. Aquatic fungi and anaerobic fungi are the other candidates for bioremediation.
Among other fungi used in bioremediation, the yeasts, e.g., Candida tropicalis, Saccharomyces cerevisiae, S. carlbergensis and Candida utilis are important in clearing industrial effluents of unwanted chemicals. Agaricus bisporus and Lentinus oloides are important in lignocellulose decomposition. Corius versicolor is important in cleaning up pulp and paper mill wastes. Consortia of fungi and bacteria (usually uncharacterised) are used in composting, the most useful waste disposal practice. Phenolic azo dyes have been shown to be oxidized by the enzyme laccase produced by Pyricularia oryzae( Chivukula and Renganathan, 1995).
Future outlook
Whenever bioremediation figures as the topic of discussion, bacterial agents come into focus and fungi are much less studied. One should realize, however, the greater potential of fungi by virtue of their aggressive growth, greater biomass production and extensive hyphal reach in soil. More research will be focused in future on using the diverse fungal flora for bioremediation. The work on the microbial diversity in the electroplating industrial effluents is going on in our laboratory. There are several promising fungi that can degrade zinc, cyanide and chromium in these effluents.
Future work will be more focussed on the biotechnological aspects. It may be possible to clone the highly efficient degradative enzyme producing genes into bacteria and conversely, bacterial genes can be transferred to fungi which are suitable. The high surface-to-cell ratio of filamentous fungi makes them better degraders under certain niches like contaminated soils. Fungi have been shown to even solubilize partially coal, a highly polymeric substance more complex than lignin. There is no doubt, therefore, regarding fungi being harnessed more and more in environmental bioremediation work in future.
Literature cited
Alexander, M 1994. Biodegradation and Bioremediation. Acad. Press, San Diego, Calif.
Ashoka, C., Manjunath, K. and Sullia, S.B. 2000. Biological treatment of combined textile dye effluent. In : Ecology of Fungi, D.J.Bhat and S. Raghukumar (eds.), 63-67.
Ashoka, C. , Geetha, M.S. and Sullia, S.B. 2002. Bioleaching of composite textile dye effluent using bacterial consortia. Asian Jr. Microbial Biotech. Env. Sci.,4:65-68.
Atlas, R.M. and Unterman, R. 1999. Bioremediation. In: Industrial Microbiology & Biotechnology, 2nd Ed. , ASM Press, Washington, 666-681
Barr, D.P. and Aust, S.D. 1994. Mechanisms the white rot fungi use to degrade pollutants. Env. Sci. Technol., 28: 79A-87A.
Bennett, J.W. and Faison, B.D. 1997. Use of Fungi in Biodegradation. In: Environmental Microbiology, ASM Press, Washington.
Carroll, G.C. and Wicklow, D.T. 1992. The Fungal Community. Its Organization and Role in the Ecosystem. 2nd ed. Marcel Dekker Inc., N.Y.
Chivukula, M. andRenganathan, V. 1995. Phenolic azo dye oxidation by laccase from Pyricularia oryzae. Appl. Environ. Microbiol., 61:4374-4377.
Cooke, R.C. and Rayner, A.D.M. 1984. Ecology of Saprophytic Fungi. Longman, London.
Davis, M.W., Glaser, J.A., Evans, J.W. and Lamar, R.T. 1993. Field evaluation of lignin degrading fungu Phanerochaete sordida to treat creosote contaminated soil. Environ. Sci. Technol., 27: 2572-76.
Frankland, J.C., Hedger, N.H. and Swift, J.J.(Eds.) 1982. Decomposer Basidiomycetes. Their Biology and Ecology. Cambridge Univ. Press, Cambridge.
Kirk, T.K., Connors, W.J. and Zeikus, J.G. 1976. Requirement of growth substrate during lignin degradation by two wood rotting fungi., Appl. Eviron. Microbiol., 32: 192-194.
Lamar, R.T. Evans, J.W. and Glaser, J. 1993. Solid phase treatment of pentachlorophenol-contaminated soil using lignin degrading fungi. Environ. Sci. Technol., 27: 2566-71.
Nigam, P., Banat, I.M., McMullan, G., Dalel Singh, and Marchant, R. 1995. Microbial degradation of textile effluent containing Azo, Diazo and reactive dyes by aerobic and anaerobic bacterial and fungal cultures. 36th Annu. Conf, AMI, Hisar, 37-38.
Pauli Ollikka, Kirsi Alhonmaki, Veli-Matti Leppanen, Tuomo Glumoff, Timo Raigola and Hari Suominen 1993. Decolorization of Azo Triphenyl Methane Heterocyclic and Polymeric dyes by lignin peroxidase, isoenzymes from Phanerochaete chrysosporium. Appl. Environ. Microbiol., 59: 4010-4016.
Rose, A.H. 1981. Microbial Biodeterioration. Vol 6, Economic Microbiology. Academic Press, London.
Sami and Radhaune 1995. Role of lignin peroxidase and manganese peroxidase from Phanerochaete chrysosporium in the decolorization of olive mill waste water. Appl. Envron. Microbiol., 61: 1098-1103.
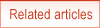
|
|